The seasonal environment of lake systems within the Northern Hemisphere can affect the regional climate regime and weather events. Ice cover energy balance drives lake ice growth and decay. Atmospheric circulation, precipitation and incoming solar radiation affect the lake energy balance. The energy balance is also dependent on the physical lake dimensions, surrounding topography and geographic location. Annual lake ice freeze-up is driven by climate regimes between October to November in the circumpolar Arctic. Snow accumulation increases lake ice thickness; wind stress can lead to delayed freeze-up and early break-up by reducing the structural integrity of the lake ice. In the spring, increased wind can also induce melting by driving the interaction between the warming atmosphere and cool ice surface. Melt is also a factor of lake depth and therefore its heat storage capacity; larger lakes undergo seasonal changes at a slower rate than smaller, shallow lake systems. Additionally, lakes situated at a high elevation usually have a longer ice season. The inflow from river systems can effect lake cover by mixing, inputting heat and creating water flow, resulting in earlier ice creak up seen adjacent to inflow regions. The seasonal processes of Arctic lakes are discussed below (Brown & Duguay, 2010; SWIPA, 2011; DeBeer et al., 2016).
Freeze-Up
The annual winter freeze-up processes of Arctic lake systems are a result of the regional climate regime during the ice-free (summer to fall) months. Warmer temperatures and more absorbed solar radiation can reduce freeze-up capabilities of a lake. However, larger lakes generally have a higher heat capacity and are not as readily affected by high temperature and radiation (Brown & Duguay, 2010; SWIPA, 2011).
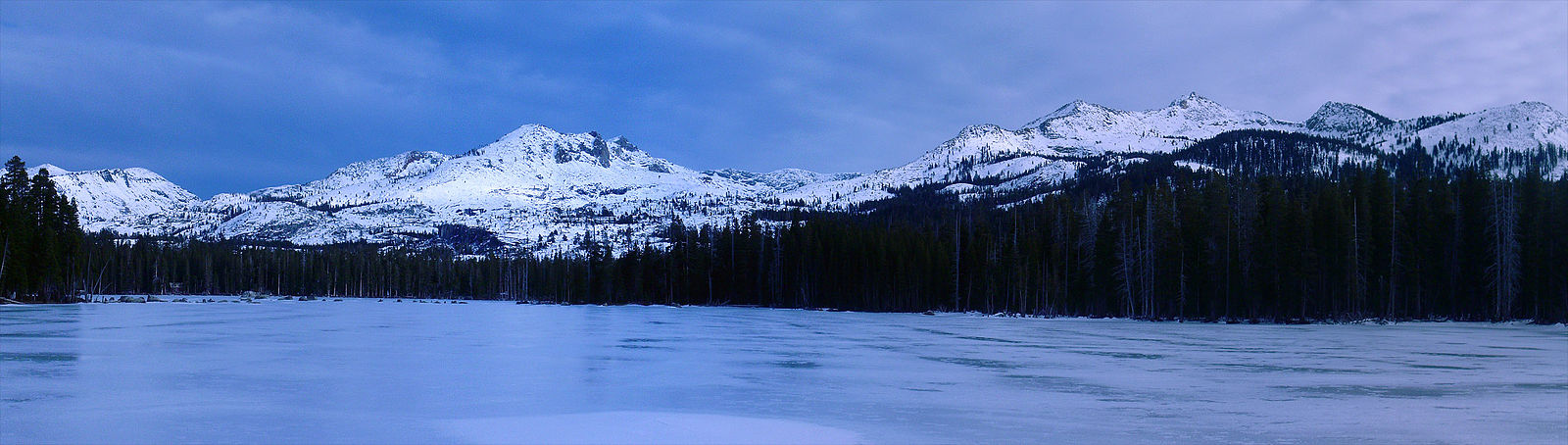
At the commencement of freeze-up, thin skim ice forms along the lake surface. Often, early skim ice development is hindered by physical wind stress. As a consequence, early ice formation processes may have to occur multiple times before solid ice is generated. Generally, ice first develops around the edges of a lake, where most heat has been lost to the atmosphere. As this ice becomes solid, and is generated across the whole lake surface, it is clear in appearance (congelation ice). As this ice continues to grow in depth, the lake below becomes insolated from the surrounding cool atmosphere. The interaction between the cool atmosphere and lake ice surface allows continuous ice growth (Brown & Duguay, 2010; SWIPA, 2011).
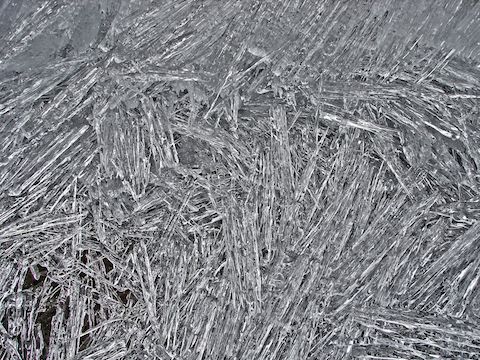
Ice Growth
Factors affecting the ice growth rate of lake ice influence precipitation and temperature. The underlying water is protected from the surrounding cold atmosphere, since lake ice has an insolating effect. For this reason, as the season progresses, heat is lost through the ice surface, gradually increasing ice depth. When snow accumulates on the top of lake ice surfaces, this process decelerates since the snow acts as an insulator to the ice below. However, in distinctive circumstances, snow cover can contribute to ice growth. In this situation, snow must have substantially lowered ice level by straining the surface, flooding the snow cover. Once the snow cover is flooded, it can readily freeze into ice, called white ice. This ice is very light in colour, and can increase the amount of solar radiation reflection by reason of its high albedo (Brown & Duguay, 2010; SWIPA, 2011).
Clouds are also a factor in ice growth processes; two general influential processes are the reflection of solar radiation back into the upper atmosphere and the entrapping of heat in the troposphere. Both of these processes are continuously occurring, however, the extent to which each occurs is important for the lake ice system. If the process of reflection is stronger, the lake will experience a cooling effect. If the heat trap process is stronger, the lake will experience a warming effect. However, during the absence of solar influence, during high Arctic deep winter, the deficiency of cloud cover is most beneficial to ice growth. Without incoming shortwave radiation, outgoing longwave radiation from the ground surface is the dominant mode of heat transport. Without clouds, this heat can be released high into the atmosphere, cooling the regional environment, encouraging ice development (Brown & Duguay, 2010; SWIPA, 2011).

Ice Break-Up
As the Arctic season changes from winter to spring, the atmosphere warms, inducing lake ice decay. Changes in water input properties, precipitation and wind circulation are important factors in this seasonal change. Vertically, the break-up begins with the upper snow layers, and continues through the ice layers as well as along the water-ice boundary. Horizontally, the break-up begins along marginal lake regions. Both mechanical (water input, wind stress) and thermal stress are effective at disintegrating the ice crystals. In some regions of the Arctic, usually at the highest latitudes, lake ice will only partially decay during the summer season. In these regions, melt ponds are apparent on the lake surface, but the lake ice continues perennially (Brown & Duguay, 2010; SWIPA, 2011).
Material on this page was provided by Maren Pauly and Tristan Mills, Department of Geography, University of Waterloo. Hover over images for photograph credit.